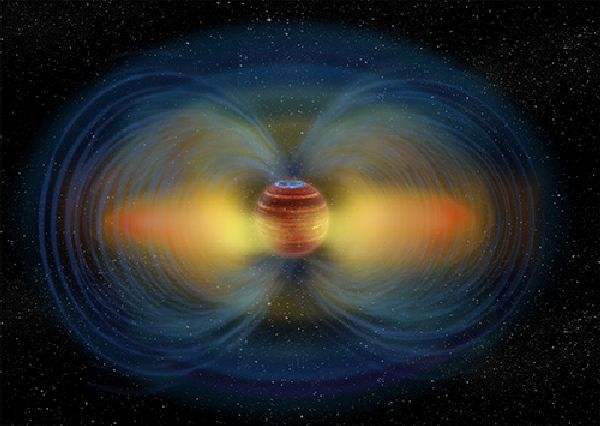
Radiation belts are zones of charged particles, primarily electrons and protons, trapped by a planet’s magnetic field.
They are typically found around planets with a significant magnetic field, such as Earth or Jupiter.
These belts produce various radiations, including radio emissions, which can be detected and studied by radio telescopes.
Lately, with the help of coordinated array of 39 radio dishes, astronomers have traced the first radiation belt beyond our solar system. The radio telescopes spanned from Hawaii to Germany.
Through the acquisition of high-resolution images, these scientists have captured persistent and intense radio emissions emanating from an ultracool dwarf.
This cloud takes on a double-lobed structure quite like the radiation belts found around Jupiter.
Magnetic bubble beyond our solar system
Melodie Kao, a postdoctoral fellow at UC Santa Cruz said that they are actively capturing images of the magnetosphere of their target by studying the radio-emitting plasma. This corresponds to the radiation belt within the magnetosphere.
For the first time, such observations have been conducted on an object the size of a gas giant planet located beyond our solar system.
Planets with strong magnetic fields are surrounded by a magnetosphere, which creates a protective “magnetic bubble” around them.
The magnetosphere can capture and accelerate particles to high speeds, approaching the speed of light.
In our solar system, all planets with magnetic fields, such as Earth, Jupiter, and the other giant planets, have radiation belts containing these high-energy charged particles trapped by the planet’s magnetic field.
For instance, the Van Allen belts, also known as Earth’s radiation belts, are two donut-shaped regions. The belt comprises of high-energy particles, which are captured by the Earth’s magnetic field from the solar wind.
While, most of the high-energy particles in Jupiter’s radiation belts come from the volcanic activity on its moon, Io.
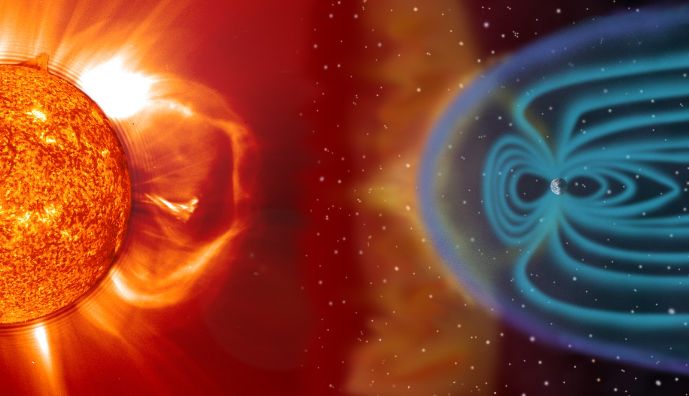
The observed radiation belt is significantly more luminous
According to Melodie Kao and her team’s recent discovery, the radiation belt they imaged – of the ultracool dwarf – is incredibly bright. Roughly, it is about 10 million times brighter than Jupiter’s radiation belts.
If the two radiation belts were to be compared side by side, the observed radiation belt would be significantly more luminous.
Similar physics inside low-mass stars to brown dwarfs and gas giant planets
In the current research, the ultracool dwarf lies on the boundary between low-mass stars and massive brown dwarfs.
The physics inside this range of masses, which connects low-mass stars to brown dwarfs and gas giant planets, is very similar, even though their formation processes can be different.
As Kao explains, this “mushy part” of the mass continuum provides a fascinating area of study for understanding the similarities and differences between stars, brown dwarfs, and gas giant planets.
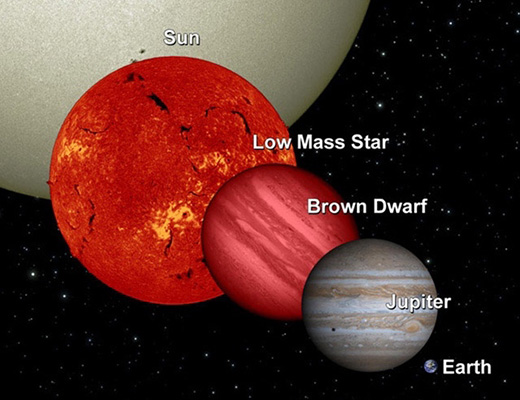
Strength and shape of the magnetic field
Theoretical knowledge and numerical models might assist in predicting the given set of input data. However, Kao suggests that understanding the strength and shape of the magnetic fields around the current type of object is an area of exploration that is yet to be fully explored.
For instance, auroras can provide insights into the strength of a planet’s magnetic field. But they cannot provide information on its shape. Therefore, the study offers innovative approach to assess the shape of magnetic fields surrounding brown dwarfs and exoplanets.
Why does the shape of a planet’s magnetic field important?
The strength and shape of a planet’s magnetic field can have a significant impact on its habitability.
It helps in maintaining favourable atmosphere and climate. After all, stable environment is an important consideration in assessing the potential habitability of exoplanets.
For a planet to have a magnetic field, its interior must be sufficiently hot to generate electrically conducting fluids.
In the case of Earth, the molten iron in its core serves as the conducting fluid, while in Jupiter, the conducting fluid is metallic hydrogen under high pressure.
According to Kao, metallic hydrogen is also likely responsible for generating magnetic fields in brown dwarfs, while ionized hydrogen serves as the conducting fluid in the interiors of stars.
The ultracool dwarf LSR J1835+3259
According to Kao, the ultracool dwarf LSR J1835+3259 was the only object in this study that could provide high-quality data to resolve its radiation belts.
The mentioned ultracool dwarf can serve as a valuable case to demonstrate how steady-state, low-level radio emissions can indicate the presence of radiation belts in the large-scale magnetic fields of such objects.
With this understanding, when similar radio emissions are detected from brown dwarfs and eventually gas giant exoplanets, scientists can be more confident in inferring the presence of significant magnetic fields, even if the telescopes used may not have the resolution to visualize the shape of those fields.
Kao expressed excitement about the Next Generation Very Large Array, which is a project undertaken by the National Radio Astronomy Observatory (NRAO). With the new tool in hand, astronomers expect to surface many more extrasolar radiation belts in the near future.
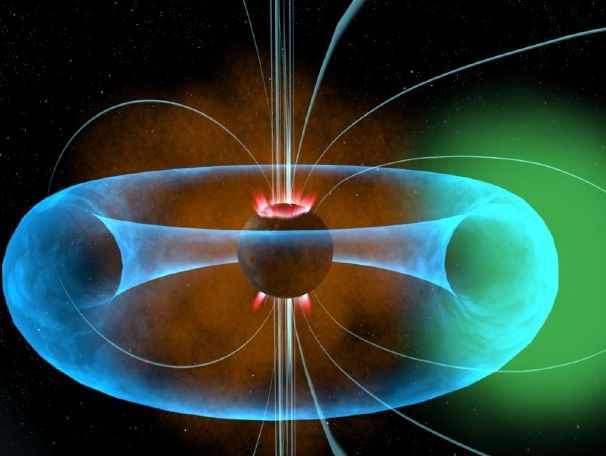
Takeaway
The recent discovery of the radiation belt around the ultracool dwarf, and the resulting images, provides a new and exciting opportunity for planetary scientists to evaluate and refine their theoretical models.